Second Brain is helping to lead in the era of integrative whole body treatments to treat ailments.
We are increasingly realizing that different systems of the body are interconnected and cannot be completely understood in isolation. The brain-gut connection is one very important example of this phenomenon.
The study of the microbiome has in recent years undergone a period of expansion that translated into a significant increase in our understanding of its role in health and disease.
The intestines are closely tied into brain health because they perform and important security function, much like the blood-brain barrier (BBB) protects your brain from harmful compounds in your blood. The intestines are vital for regulating which components from the foods and drinks you consume are absorbed into the blood stream and possibly into your brain much like a filter. When the intestines are inflamed or damaged, it creates a break in this security system allowing for the absorption of potentially toxic compounds and even microorganisms like bacteria and viruses to bypass the security filter and enter directly into the blood stream and ENS. Once these compounds have entered your blood stream and ENS, they are one step closer to impacting the health of other organs including your brain. Source: https://pinnaclife.com/the-gut-brain-connection-protecting-your-second-brain/
Second Brain utilizes precise bioelectric signaling sequences delivered to the gut microbiota, the nervous system and brain to boost immune system strength and to help modulate brain disorders related to an un-healthy balance of key related brain mood and function related protein expressions such as serotonin.
Enteric nervous system
Technically known as the enteric nervous system, the second brain consists of sheaths of neurons embedded in the walls of the long tube of our gut, or alimentary canal, which measures about nine meters end to end from the esophagus to the anus. The second brain contains some 100 million neurons, more than in either the spinal cord or the peripheral nervous system.
In studies, David Geffen School of Medicine at the University of California, Los Angeles (U.C.L.A.) scientists were shocked to learn that about 90 percent of the fibers in the primary visceral nerve, the vagus, carry information from the gut to the brain and not the other way around.
The enteric nervous system uses more than 30 neurotransmitters, just like the brain, and in fact 95 percent of the body’s serotonin is found in the bowels. Because antidepressant medications called selective serotonin reuptake inhibitors (SSRIs) increase serotonin levels, it’s little wonder that meds meant to cause chemical changes in the mind often provoke GI issues as a side effect. Irritable bowel syndrome—which afflicts more than two million Americans—also arises in part from too much serotonin in our entrails, and could perhaps be regarded as a “mental illness” of the second brain.
Scientists are learning that the serotonin made by the enteric nervous system might also play a role in more surprising diseases: In a new Nature Medicine study published online February 7, a drug that inhibited the release of serotonin from the gut counteracted the bone-deteriorating disease osteoporosis in postmenopausal rodents. (Scientific American is part of Nature Publishing Group.) “It was totally unexpected that the gut would regulate bone mass to the extent that one could use this regulation to cure—at least in rodents—osteoporosis,” says Gerard Karsenty, lead author of the study and chair of the Department of Genetics and Development at Columbia University Medical Center.
Serotonin seeping from the second brain might even play some part in autism, the developmental disorder often first noticed in early childhood. Gershon has discovered that the same genes involved in synapse formation between neurons in the brain are involved in the alimentary synapse formation. “If these genes are affected in autism,” he says, “it could explain why so many kids with autism have GI motor abnormalities” in addition to elevated levels of gut-produced serotonin in their blood.
Source: Scientific American Feb. 2010 https://www.scientificamerican.com/article/gut-second-brain/
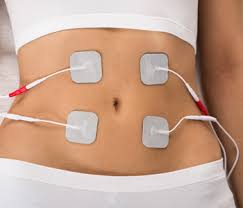
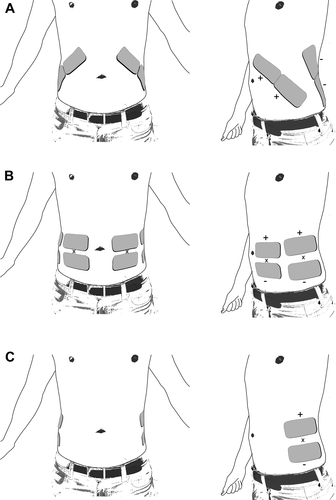
- For improving immune system strength.
- For treating multiple brain disorders including anxiety, depression, drug addiction, excessive anger bouts, violent behavior, bi-polar disorder or other forms of excessive mood swings.
- For treating multiple gastrointestinal diseases such as irritable bowl syndrome and inflammatory bowel disease such as ulcerative colitis.
- For treating obesity.
- For treating osteoporosis and other bone mass disorders.
- For treating cancer.
- For treating autism.
- For treating Alzheimer’s.
- For treating Parkinson’s.
- For treating neurodegeneration in all forms.
- For treating chronic and acute inflammation – Click here
- For treating multiple sclerosis
- For treating psoriasis.
- For treating rheumatoid arthritis.
- For reducing stroke risk.
- For reducing cardiovascular disease risk.
A New Connection between the Gut and Brain
A surprising way that diet leads risks of stroke and cognitive impairment
Source: Click Here
Now, new research shows another connection: immune signals sent from the gut can compromise the brain’s blood vessels, leading to deteriorated brain health and cognitive impairment. Surprisingly, the research unveils a previously undescribed gut–brain connection mediated by the immune system.
The implications of this newly identified gut–brain connection extend to several autoimmune disorders, including multiple sclerosis, rheumatoid arthritis, psoriasis, and inflammatory bowel disease, that have been shown to activate the same immune signaling pathway implicated in this study. These autoimmune disorders have a high stroke risk and are linked to poorly functioning blood vessels in the nervous system.
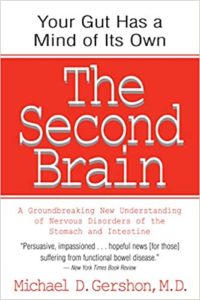
Enteric nervous system
The enteric nervous system (ENS) or intrinsic nervous system is one of the main divisions of the autonomic nervous system (ANS) and consists of a mesh-like system of neurons that governs the function of the gastrointestinal tract.[1] It is capable of acting independently of the sympathetic and parasympathetic nervous systems, although it may be influenced by them. The ENS is also called the second brain.[2][3]It is derived from neural crest cells.[4][5]
The enteric nervous system is capable of operating independently of the brain and spinal cord,[6] but does rely on innervation from the autonomic nervous system via the vagus nerve and prevertebral ganglia in healthy subjects. However, studies have shown that the system is operable with a severed vagus nerve.[7] The neurons of the enteric nervous system control the motor functions of the system, in addition to the secretion of gastrointestinal enzymes. These neurons communicate through many neurotransmitters similar to the CNS, including acetylcholine, dopamine, and serotonin. The large presence of serotonin and dopamine in the gut are key areas of research for neurogastroenterologists\
Structure.
The enteric nervous system in humans consists of some 500 million neurons[11] (including the various types of Dogiel cells),[1][12] 0.5% of the number of neurons in the brain, five times as many as the one hundred million neurons in the human spinal cord,[13] and about 2/3 as many as in the whole nervous system of a cat. The enteric nervous system is embedded in the lining of the gastrointestinal system, beginning in the esophagus and extending down to the anus.[13]
The neurons of the ENS are collected into two types of ganglia: myenteric (Auerbach’s) and submucosal (Meissner’s) plexuses.[14] Myenteric plexuses are located between the inner and outer layers of the muscularis externa, while submucosal plexuses are located in the submucosa.
The Second Brain Aging
The Second Brain Aging: Linking Gut Microbiota to Neurodegeneration
Aging is a process characterized by progressive functional decline of all physiological systems. In the gastrointestinal tract, aging involves the degeneration of enteric nervous system (ENS), alterations in gastrointestinal motility, perturbations in small intestinal permeability and mucosal defense system, which may promote the development of gastrointestinal diseases, affect the local and systemic inflammatory status, and deeply influence both the composition and function of resident microbiota (87–89).
Aging is also associated with broad changes in brain and whole body physiology that may influence gut microbiota–brain axis. In particular, the HPA axis is deeply perturbed, through a self-reinforcing cycle mediated by the hyperactivation of the HPA axis that leads to increased basal glucocorticoid release and the impaired HPA negative feedback due to reduced central glucocorticoid receptor expression (90, 91). HPA axis dysfunctions have been associated with decline in hippocampal volume and cognitive performance, and increased risk of late-life depression and anxiety (92, 93). Also circadian rhythm disruption, which is typical of aging, may be involved in this process, due to the potential effect on both cortisol level fluctuations and gut microbial activities (94, 95).
The aging brain is also deficient in the synthesis of neurotrophic factors, including BDNF (96) as well as several neurotransmitters, including 5-HT and DA, all of which lead to neuronal and cognitive dysfunction (97, 98). BBB breakdown is an early event in the aging human brain that begins in the hippocampus and may contribute to cognitive impairment (99).
Aging is also characterized by the progressive decline in immune function (immunosenescence) associated with a chronic, low-grade inflammation (inflamm-aging) (100, 101). Both processes may have many effects on the CNS, such as microglial activation, BBB breakdown, and increase in oxidative damage that may contribute to neurodegenerative and neuropsychiatric diseases (100). Remarkably, recent data suggest that, in old mice, gut microbiota contribute to inflamm-aging, and that this inflammatory phenotype may be transferred to young GF mice (102).
Major taxonomic shifts and a consistent decrease in microbial richness and diversity have been reported in people 65 years of age and older and these changes were associated with worsening of health status and frailty (89, 103). Similar findings were also obtained in mice (104).
The characterization of gut microbiota of centenarians revealed the presence of significant compositional differences across life stages till extreme ages (105). In particular, core microbiota (mostly composed by the members of Ruminococcaceae, Lachnospiraceae, and Bacteroidaceae families) seem to accompany human life, decreasing in abundance along with aging (105). In longevity and extreme longevity, an enrichment in some subdominant health-associated groups (e.g., Akkermansia, Bifidobacterium, and Christensenellaceae) occurs, even with the support of some opportunistic and allochthonous bacteria (105).
Recently, the effects of aging on the microbiota gut–brain axis were assessed in male mice (106). Aged mice showed significant shifts in gut microbiota that were associated with deficits in spatial memory and increases in anxiety-like behaviors compared with young adult mice (106). These changes were positively correlated with the abundance of bacteria from the Porphyromonadaceae family. Aged mice also exhibited increased gut permeability that was associated with elevations in peripheral pro-inflammatory cytokines (106).
These preliminary findings suggest that age-related changes in gut microbiota may impact behavioral and cognitive functions and support the relevance of the alteration in gut permeability and peripheral inflammation in mediating these effects.
As outlined earlier, the possible link between early gut microbiota–brain interactions and late onset neurological conditions, including AD and PD, is an intriguing area of research (15).
Alzheimer’s Disease
In AD, the most common form of age-related dementia, deposition of protein aggregates composed of amyloid-β (Aβ) peptide and tau in brain tissues impairs cognitive function (107). Both host- and environmental factors that regulate these processes have been described, including a potential role for gut microbiota (108)
FIGURE 2
www.frontiersin.org
Figure 2. Age-related changes in gut–brain axis possibly involved in neurodegeneration. Abbreviations: AD, Alzheimer’s disease; PD, Parkinson’s disease.
In AD, reduced microbial richness and diversity were observed, with low abundance of Firmicutes and Bifidobacterium and increased Bacteroidetes that characterized the microbiome of AD patients (108). Correlations were found between the levels of Bacteroides, Turicibacter, and SMB53 and the concentration of glial activation biomarkers in cerebrospinal fluid of AD (108).
An increase in the abundance of the pro-inflammatory Escherichia/Shigella taxon, and a corresponding reduction in the anti-inflammatory E. rectale was associated with higher levels of inflammatory mediators in patients with cognitive impairment and brain amyloidosis (109). Also in a mouse model overexpressing amyloid precursor protein and presenilin 1 (APPPS1), a distinct microbial signature was observed with an increase in Rikenellaceae and decreased Allobaculum and Akkermansia compared with age-matched wild-type controls (110).
Interestingly, reduced levels of Akkermansia characterize gut microbiota of mice with obesity and type 2 diabetes (111), two potentially modifiable risk factors for AD (107). Importantly, both young and old GF APPPS1 transgenic mice displayed a drastic reduction of cerebral Aβ pathology when compared with control mice, along with a reduced microgliosis (110). Further to this, colonization of GF-APPPS1 transgenic mice with microbiota from conventionally raised APPPS1 transgenic mice increased cerebral Aβ pathology, while colonization with microbiota from wild-type mice was less effective in increasing cerebral Aβ levels (110). Notably, GF-APPPS1 displayed increased levels of the Aβ-degrading enzymes insulin degrading enzyme and neprilysin degrading enzyme, suggesting a mechanism through which gut microbiota influence cerebral Aβ amyloidosis (110).
In the same mouse model of AD, life-long antibiotic treatment induced a considerable perturbation in gut microbial composition (including an expansion of Akkermansia) that was associated with marked changes in the circulating cytokine/chemokine network, a striking reduction in amyloid plaque deposition, and a concomitant increase in soluble Aβ (112). This was accompanied by alterations in neuroinflammatory milieu that lead to reduced plaque-localized gliosis and altered microglial morphology (112). Remarkably, early post-natal antibiotic treatment alone resulted in long-term alterations in gut microbial genera that were associated with changes in the inflammatory environment of serum and cerebrospinal fluid and attenuated Aβ amyloidosis in a manner similar to that observed in mice subjected to life-long antibiotic selection pressure (113). These findings corroborate the hypothesis of the presence of critical developmental periods in which the commensal microbiota manipulation may have long-lasting effects on host immunity and potential implications for neurodegenerative diseases.
In another model of AD, the 5xFAD transgenic mouse, elevated levels of APP were found not only in the brain but also in the different gut districts and this was associated with a distinct fecal microbiota profile relative to wild-type animals, with an increase in pro-inflammatory species (e.g., Clostridium leptum) (114).
Alterations in gut microbiota composition together with the increase in intestinal permeability with age may lead to the translocation of microbes or microbial components [i.e., lipopolysaccharide (LPS)] from the gut to induce systemic and CNS inflammation (115). Interestingly, in vitro and in vivo studies have demonstrated a possible association between LPS and AD pathology. Coincubation of Aβ peptide with LPS potentiated amyloid fibril formation (116), and systemic administration of LPS in wild-type and transgenic AD mice induced neuroinflammation, amyloid deposition, and tau pathology (117–119). Moreover, in postmortem brain parenchyma and blood vessels from patients with AD, levels of LPS and Gram-negative E. coli fragments were greater compared with control brains and colocalized with amyloid plaques (120).
While the study of the microbiota gut–brain axis in AD is still in its infancy, promising preclinical data suggest that the modulation of gut microbiota through dietary ingredients or probiotics may provide a means to counteract the development or progression of neurodegenerative disease. For instance, in a triple-transgenic mouse model of AD (3xTg-AD), a formulation of lactic acid bacteria and bifidobacteria changed the composition of gut microbiota, stimulated the production of beneficial metabolites (e.g., increased SCFAs), reduced the levels of pro-inflammatory cytokines, increased gut hormones concentration and positively modulate quality control processes and proteolysis, reducing Aβ load and improving cognitive function (121). Moreover, the administration of the probiotic mixture VSL#3 to aged rats induced a robust perturbation in gut microbiota composition, that was accompanied by gene expression changes in the brain cortex, attenuated age-related deficits in long-term potentiation, decreased microglial activation, and increased BDNF and synapsin levels (122). In addition, 3-hydroxybenzoic acid and 3-(3-hydroxyphenyl)propionic acid, the phenolic products of microbial conversion of grape seed polyphenol extracts (and other dietary polyphenols), may potently interfere with the assembly of Aβ peptides into neurotoxic Aβ aggregates in vitro (123).
Despite these interesting preliminary findings, more work is needed to determine whether gut microbiota modulation may be employed for the prevention and/or treatment of AD pathogenic processes.
Parkinson’s Disease
Parkinson’s disease is the second most common neurodegenerative disorder, affecting 2–3% of the population ≥65 years of age (124, 125). Degeneration of the dopaminergic nigro-striatal pathway and widespread intracellular α-synuclein accumulation are the neuropathological hallmarks of PD that are associated with bradykinesia and other cardinal motor and non-motor features (126).
Gastrointestinal dysfunction, in particular in the form of constipation, is among the most frequent prodromal non-motor symptoms of PD that may precede motor symptoms by decades (126). At later disease stages, oral issues including drooling and swallowing problems and delays in gastric emptying further exacerbate gastrointestinal dysfunction (127). Aggregates of α-synuclein have been retrieved in the mucosal and submucosal nerve fibers and ganglia of the ENSs of PD patients at early disease stages (128, 129). In addition, some observations from experimental models support the intriguing hypothesis that intestinal α-synuclein may spread to the brain via postganglionic enteric neurons and the vagus nerve (130). Interestingly, the risk of developing PD was significantly decreased in patients who underwent a full truncal vagotomy compared with those who underwent selective vagotomy and in the general population (131).
Not surprisingly, gastrointestinal disturbances in people with PD are accompanied by alterations in fecal and mucosal microbial populations (31, 132–134). In particular, a reduced abundance of Prevotellaceae, mucin producers that regulate intestinal permeability, was commonly reported in PD patients (31, 132, 135, 136), while Enterobacteriaceae were positively associated with the severity of postural instability and gait difficulty (31). Clostridium coccoides group was high in early PD patients, while Lactobacillus gasseri subgroup was high in advanced PD patients (132). A pro-inflammatory dysbiosis, characterized by low counts of “anti-inflammatory” butyrate-producing bacteria from the genera Blautia, Coprococcus, and Roseburia and higher “pro-inflammatory” Proteobacteria of the genus Ralstonia was also reported in individual with PD (133). Individuals affected by PD also showed lower levels of SCFA concentrations, derived from host–microbiota cometabolism, that may have neuroactive and immunomodulating properties (135). Other evidence of microbiota dysregulation in PD includes small intestine bacterial overgrowth and high rates of Helicobacter pylori infection (137, 138). It is worth noting that this infection has also been involved in the pathogenesis of AD (139). Finally, the total abundance of intestinal bacterial was found to decrease during PD progression, with a low count of Bifidobacteriumassociated with worsening of PD symptoms (134).
Collectively, these findings suggest that perturbations in gut microbiota structure and function may be associated with the development and progression of PD through several potential mechanisms, including inflammation and bacterial translocation (Figure 2). However, findings in humans remain largely descriptive. Again, animal models provided some useful insights into the physiopathological mechanisms linking gut dysbiosis to PD. Under GF conditions, or when bacteria were depleted in post-natal life following antibiotic treatment, transgenic mice overexpressing α-synuclein showed reduced microglia activation, α-synuclein inclusions, gastrointestinal symptoms, and motor deficits compared with animals with a complex microbiota (140). Moreover, administration of a mixture of microbially derived SCFAs (acetate, propionate, and butyrate) restored all major features of PD in GF mice, suggesting that microbial metabolic mediators may promote microglia activation and α-synuclein aggregation and contribute to motor dysfunction in PD (140). Remarkably, mice transplanted with PD microbiota compared with mice who received microbiota from healthy human controls displayed enhanced motor dysfunction, suggesting that dysbiosis may be the environmental factor that combined with a genetic predisposition (α-synuclein overexpression) influences disease outcomes in mice (140).
As already outlined for AD, in neurodegenerative diseases, including PD, the passage of bacterial products from the intestine to the circulation and into brain, or “molecular mimicry” processes induced by bacterial amyloids may trigger a persistent neuroinflammation (28, 141, 142) that in turn contributes to neuronal dysfunction and death (143). In this scenario, it has recently been proposed that Aβ production and aggregation may originally act as an antimicrobial defense and then infectious or sterile inflammatory stimuli may drive amyloidosis (144).
While it is currently recommended the use of fermented milk containing probiotics and prebiotic fiber in PD patients with constipation (145), the possible beneficial effects of the manipulation of gut microbiota (through diet, live bacteria, or microbiota transplantation) on the initiation or progression of the neurodegenerative process have not yet been explored. Further studies are also needed to assess the possible interactions among these interventions and levodopa uptake and availability.
Source: Click Here